Our research axes
Structural Biology of Novel Targets in Human Diseases
Structural Dynamics of Proteins and RNA
Proteins are not rigid structures: they move and adapt to their environment in order to optimize their shapes and functions. We combine X-ray crystallography/Cryo-EM with in-solution NMR spectroscopy (coll. with Jensen’s Lab) to provide a full picture of the structural dynamic properties of biological macromolecules. This approach has proven to be extremely powerful to better capture the ultra-dynamic properties of macromolecules in solution which are otherwise difficult to tackle by using only crystallography or electron microscopy techniques. For example, by using this strategy we have been able to solve a long-standing question in biology: how aromatic residues in the core of proteins can fully rotate (180 degrees) while being engaged in crucial interactions that maintain protein folding and so ensure function? We have shown the structural rearrangements, known as “breathing motions”, that are required for the flipping of an aromatic residue in the core of a folded protein (Fig. 1) (Mariño Pérez et al 2022). This has implications for both protein design and structure prediction by highlighting how small changes in the protein core can lead to major structural changes, and provides novel insights into how these small alterations can lead to diseases.
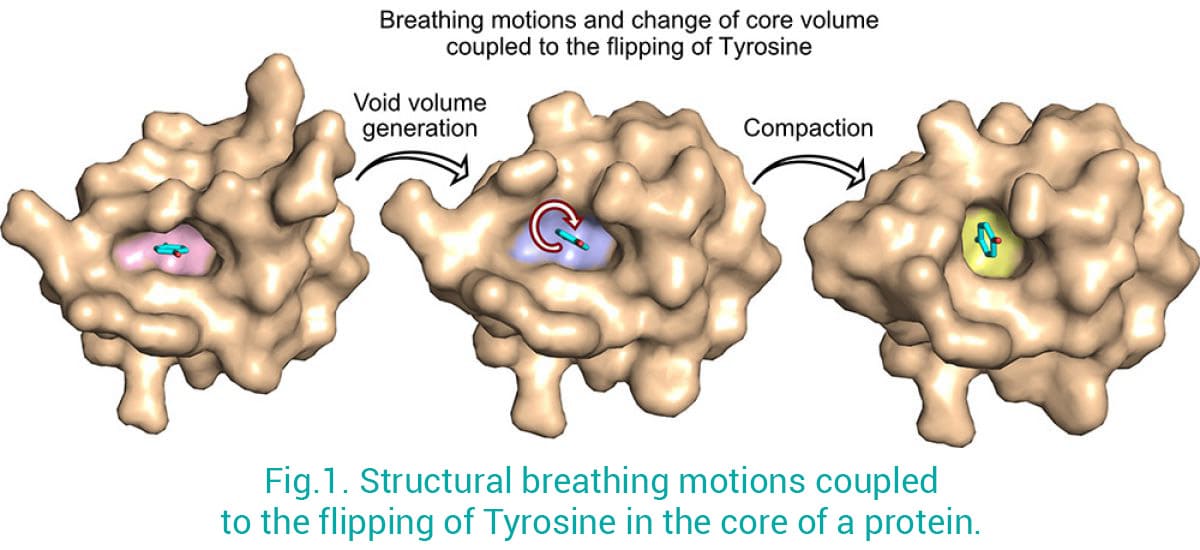
AminoAcyl-tRNA Synthetases (AARS)
One of our focuses is Leucyl-tRNA synthetases (LeuRS), which charge leucine to the cognate tRNA-Leu and prevent tRNA mischarging to chemically related amino acids. We have determined structures of LeuRS in different functional states: 1) the aminoacylation state, where the 3’-tRNA is in the synthetic site ready to accept the adenylate leu-AMP; 2) the post-transfer editing state, where the mischarged 3’-tRNA is bound into the editing site for proof-reading. This provided unprecedented insight into the structural dynamics and the translocation of the 3’-tRNA between the synthetic and the editing sites, separated more than 30 Å (Palencia et al 2012, Chopra et al 2013) (Fig. 2).
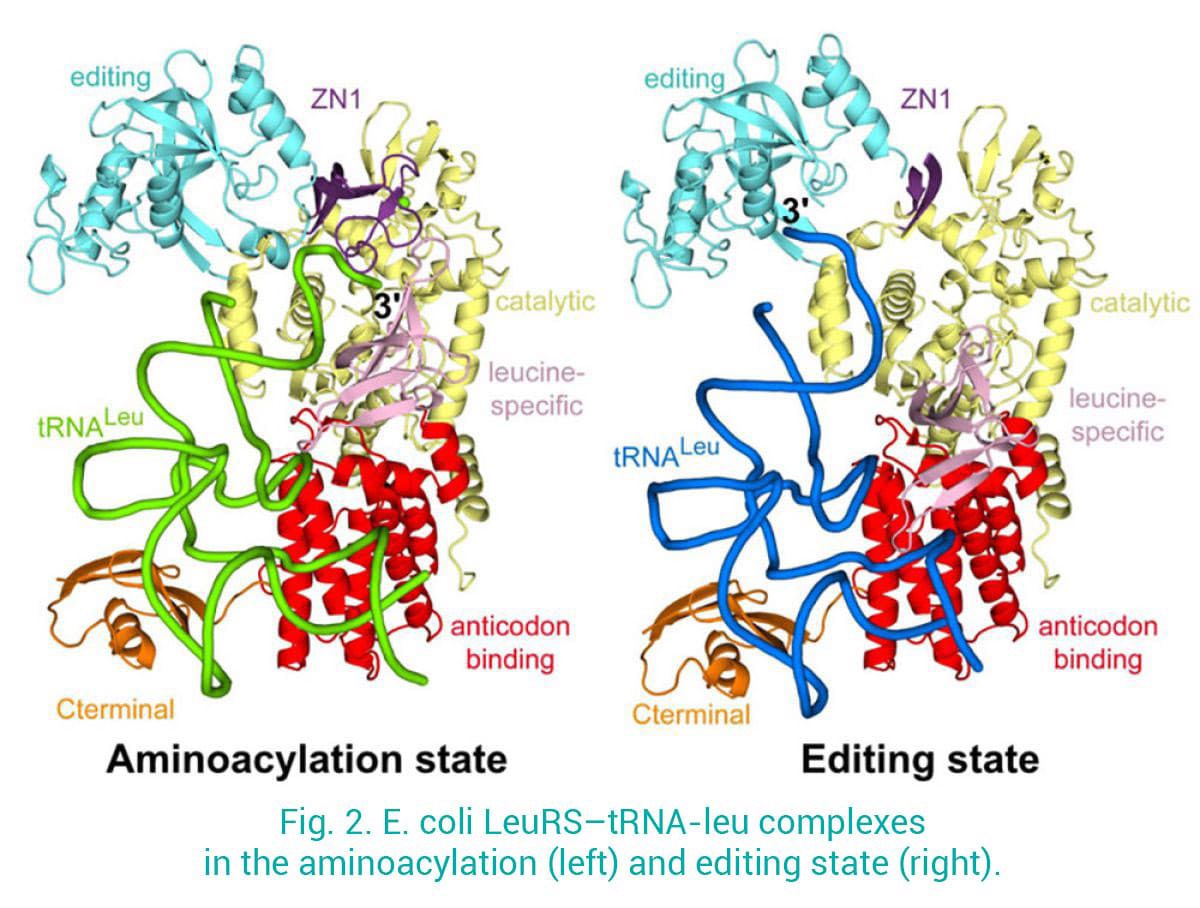
Using this mechanistic insight, in close collaboration with teams in academia and industry, we contributed to the development of antimicrobials targeting LeuRS. Our structures of LeuRS–tRNA bound to benzoxaboroles unveiled the inhibition mechanism of novel antibacterials, whereby the tRNA gets trapped at the editing site and effectively inhibits protein synthesis (Fig. 3). Using a structure-based drug design approach we brought the potency of initial hits from micro- to nano-molar and improve selectivity, allowing their progression into clinical trials. Exploiting this mechanism of action, novel anti-infectives against apicomplexan parasites were developed.
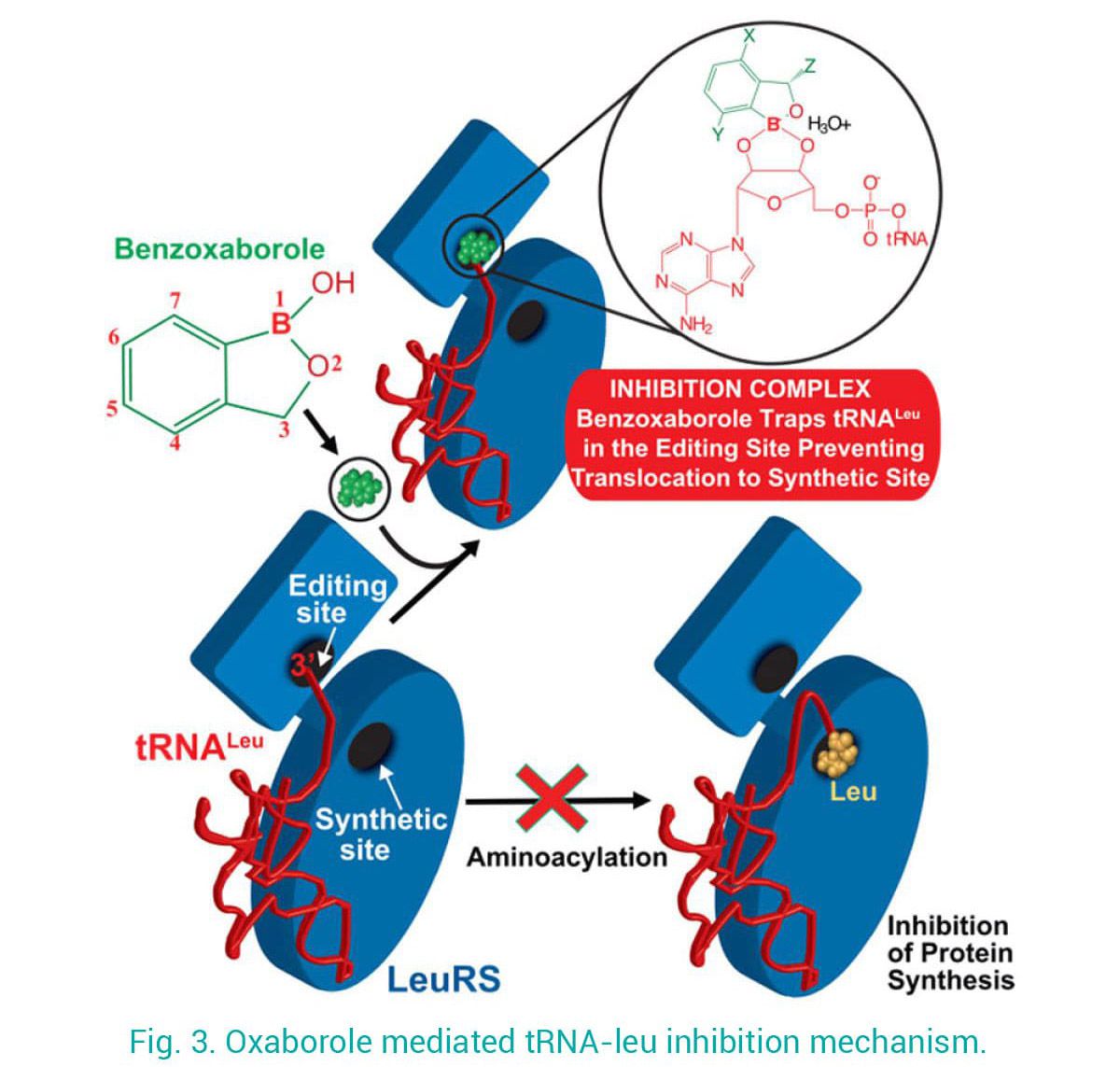
Macromolecular machineries involved in 3’-end mRNA Processing and Polyadenylation
Despite the growing evidence showing that defects in 3’-mRNA processing lead to disease, mRNA processing factors are poorly explored as drug targets. We recently showed that targeting CPSF3 by Oxaboroles in several pathogens represents an excellent approach to treat infections (Palencia et al 2017; Swale et al 2019). The homologous protein in humans, called CPSF73, associates into a large complex to cleave canonical 3’ pre-mRNAs before the addition of the poly-A tail by a polyadenosine polymerase (PAP) (Fig. 4A). CPSF73 is also recruited to a different complex involved in the maturation of replication-dependent histone pre-mRNAs (Ielasi et al 2022), which are not polyadenylated (Fig. 4B), a process tightly regulated during the cell cycle. Moreover, we have evidence that human CPSF73 is heavily upregulated in many cancers, and its overexpression correlates with poor patient prognosis. We are using an interdisciplinary approach by combining bioinformatics, cancer cell biology, structural biology and biophysical techniques to investigate the mechanisms associating CPSF73 to cancer, and the inhibition of CPSF73 by compounds as a novel therapeutic approach.
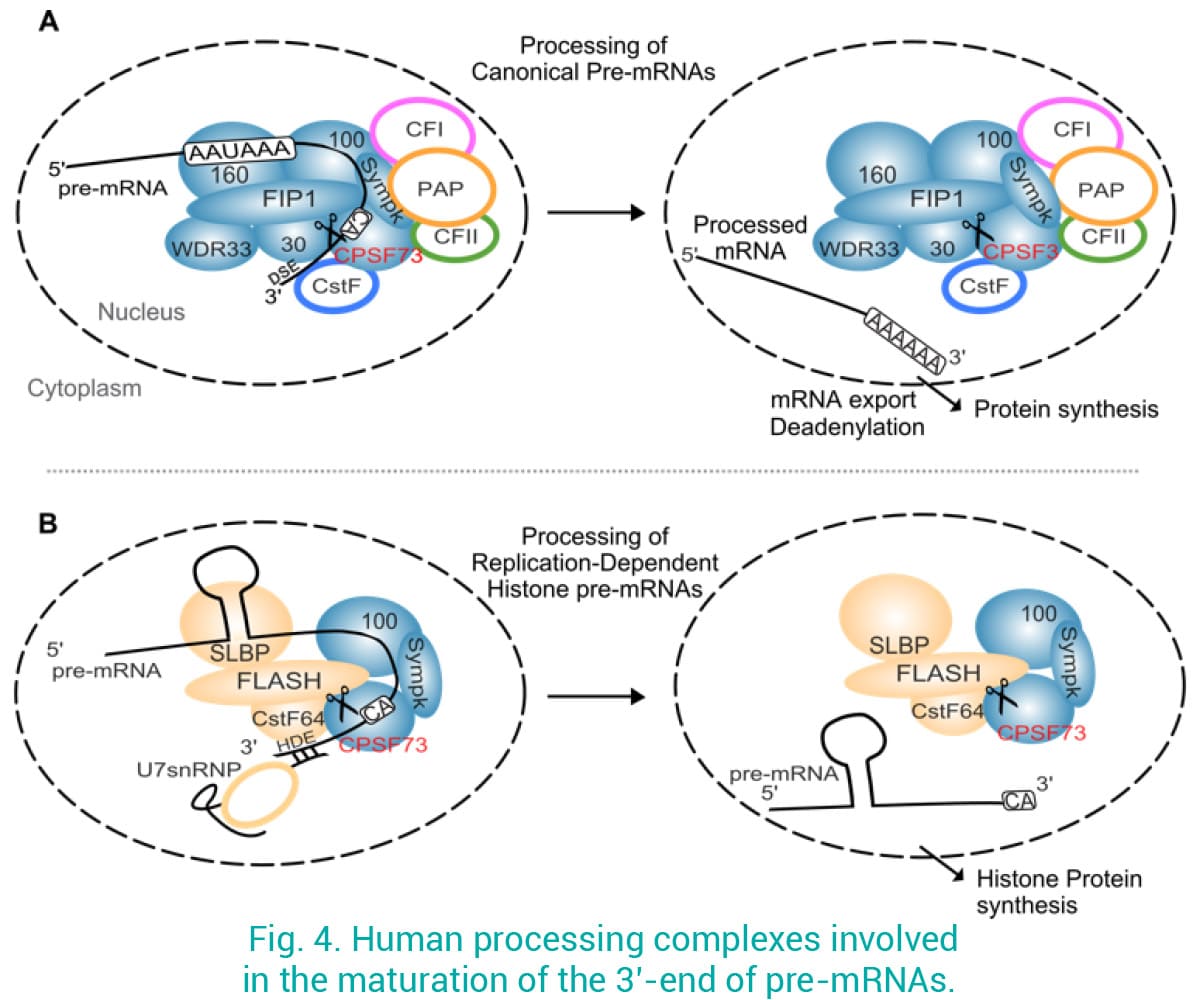
Eukaryotic complexes involved in 3’-end pre-tRNA Processing
In order to carry their functions, precursor transfer RNAs (pre-tRNAs) need to be processed into the final tRNAs that contain specific sequence and length of the 3’ and 5’ ends. This tRNA processing mechanism consists of 1) 5’ end cleavage, 2) 3’ end cleavage, 3) the addition of a the CCA acceptor motif to the 3’ end, and 4) post-processing modifications (performed in the cytoplasm).
For the few intron-containing pre-tRNAs, two additional complexes take place to enable their intron to be spliced out and the remaining part ligated. Steps 1) and 2) occur both in the nucleus or the mitochondrion depending on the tRNA isoacceptor.
We investigate nuclear pre-tRNA processing and aim at completing the limited structural data on these processes, which will be useful for the development of therapeutical applications.